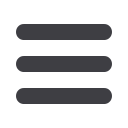

S436
ESTRO 36
_______________________________________________________________________________________________
released recently by Varian with new scan protocols. This
study aimed to investigate the influence of parameters of
the new protocols on the effective dose (E) compared to
the previous version (V1.6).
Material and Methods
Effective dose of three scan protocols (head, t horax, and
pelvis) were estimated using Monte Carlo s imulations.
BEAMnrc and DOSXYZnrc user codes were used to simulate
the OBI system integrated into a TrueBeam linac, and to
calculate organ doses resulting from the protocols
employed. Organ doses were evaluated for the ICRP adult
male and female reference computational phantoms. The
main differences between the software versions (V1.6)
and (V2.5) are: (1) the beam width was extended to 214
mm instead of 198 mm, (2) the mAs values were increased
to (150, 270, 1080) compared to (147, 267, 1056) for head,
thorax, and pelvis, respectively, and (3) the projections
number was increased to 500 for head scan compared to
367, and to 900 for thorax and pelvic scans instead of 660.
Results
The use of the scan protocols implemented in V2.5
resulted in increasing E of head scan by 13% and 12%,
where E of V1.6 was 0.27 mSv and 0.44 mSv for male and
female phantoms compared to 0.31 mSv and 0.49 mSv for
V2.5, respectively. Parameters of the new protocols, also,
led to rise E of thorax and pelvic scans by 16% and 17% for
male, respectively, and by 16% for female. E of thorax and
pelvic scans increased from 3.32 mSv and 5.95 mSv to 3.86
mSv and 6.88 mSv for male, respectively, and from 3.97
mSv and 11.38 mSv to 4.65 mSv and 13.16 mSv for female,
respectively.
Conclusion
CBCT scans play a major role in radiotherapy treatment.
The scan protocols with the new parameters were
implemented into the new software to improve the image
quality acquired with the scans, and to extend the field of
view. This helps to improve the patient positioning on the
treatment couch and deliver the specified dose to the
patient with a high accuracy, and hence optimising the
treatment output. The new head, thorax, and pelvic scans
only increased E values by 12 – 13%, 16 – 17%, and 16%,
respectively, for male and female. These increases are
acceptable when compared to improvement of the
treatment output.
PO-0815 External neutron spectra measurements for a
single room compact proton system
R. Howell
1
, E. Klein
2
, S. Price Hedrick
3
, M. Reilly
4
, L.
Rankine
5
, E. Burgett
6
1
UT MD Anderson Cancer Center Radiation Physics,
Radiation Physics, Houston- TX, USA
2
Northwell Health System, Medical Physics, Lake Success,
USA
3
Provision Center for Proton Therapy, Radiation
Oncology, Knoxville, USA
4
Washington University, Radiation Oncology, St. Louis,
USA
5
The University of North Carolina, Radiation Oncology,
Chapel Hill, USA
6
Idaho State University, Nuclear Engineering, Pocatello,
USA
Purpose or Objective
Secondary external neutrons are produced within the
physical components of the proton beam line e.g., the
double scatterer, modulation wheel, compensator, and
field aperture. In passive scattered proton therapy,
external neutrons account for a majority of neutron dose
equivalent for small fields and up to 50 % for large fields.
Spectra measurements are needed to fully and accurately
understand neutron dose equivalent from external
neutrons. Such data should be reported for proton
beamlines from each manufacturer. Here, we focused on
the single room compact proton system manufactured by
Mevion (Mevion Medical systems, Littleton, MA) whose use
is rapidly increasing in the United States and worldwide.
Material and Methods
Measurements were performed using a 250-MeV passively
scattered proton beam with a range of 20 cm, modulation
of 10 cm with the small aperture in place. Measurements
were done with a solid brass plates fully filling the
aperture opening to achieve a 'closed jaw configuration”.
This configuration was selected because it is the most
amount of high-Z material that can be in the beamline,
thus representing the maximum external neutrons
produced for the small field designation.
We performed measurements at isocenter and off axis at
40 and 100 cm from the isocenter with the gantry rotated
to 90
o
or 0
o
and couch rotated 0
o
or 270
o
, Figure 1. All
measurements were performed using an extended range
Bonner Sphere Spectrometer (ERBS). The ERBS had 18
spheres including the 6 standard Bonner spheres and 12
extended spheres with various combinations of copper,
tungsten, or lead. Each set of measurements was
performed with all 18 sphere combinations in air with the
6
LiI(Eu) scintillator. Data were unfolded using the MAXED
MXD_FC33 algorithm and normalized per unit proton Gy to
isocenter.
Figure 1:
Schematic diagram of measurement locations.
Results
The measured neutron spectral fluence at each of the six
measurement positions are shown in Figure 1. The average
energies, total fluence, and ambient dose equivalents per
proton Gy are listed in the table imbedded within figure
1. The average energy, total fluence, and ambient dose
equivalent were all highest at isocenter and decreased as
a function of distance from isocenter. While the energy
distributions for each of the fluence spectra (Figure 1)
were similar, with a high-energy direct neutron peak, an
evaporation peak, a thermal peak, and an intermediate
continuum between the evaporation and thermal peaks,
there were a higher fraction of direct neutrons at
isocenter compared to 40 and 100 cm from isocenter.
Figure 2:
Measured neutron fluence spectra at each of
measurement position. For each fluence spectrum, the
average energy, total fluence, and ambient dose
equivalent [H*(10)] are listed in the imbedded table.