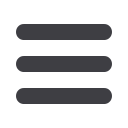

S765
ESTRO 36
_______________________________________________________________________________________________
the neutrons are produced when the electron beam hits
the tungsten target and then the primary collimation
occurs. Both the target and the primary collimator are
located at the top of the gantry head. The maximum
thermal
neutron
flux
obtained
is
3x10
5
neutrons/cm
2
.second which is higher than a standard
americium-beryllium
(Am-Be)
neutron
source.
At the isocenter plane (SSD=100 cm), the fluxes were
5.4x10
4
at the center, 1.5x10
4
at 2.5 m away and 9.9x10
3
n/cm
2
.s at the room wall which is 3.8 m away from
isocenter. The flux at the maze entrance was measured
nearly six in a ten thousand less (81 n/cm
2
.s).
Conclusion
The neutron flux distribution within the bunker was
measured with detail using 91 points. Neutron flux
distribution within the bunker found and the graph was
plotted. Thus neutron flux can found any desired point in
the room by iterations. The flux decreases as we move
away the isocenter which is compatible with the
literature. The magnitude of the neutron fluxes shows that
there is a significant amount of neutron dose within the
room. The corresponding neutron dose to the patient
however is only 0.1-0.3 % of the total dose. However,
neutrons have a high RBE and this unwanted dose is not
calculated with the TPS. The future work would be to
compare the results with the Monte Carlo simulations.
EP-1434 Comparison of small-field output factor
measurements
C. Oliver
1
, V. Takau
1
, D. Butler
1
, I. Williams
1
1
ARPANSA, Radiotherapy, Yallambie, Australia
Purpose or Objective
The Australian Radiation Protection and Nuclear Safety
Agency (ARPANSA) held a comparison in April 2016
whereby participants came to ARPANSA and measured the
output factor of a 5 mm cone . The goal of the comparison
was to compare the consistency of the small-field output
factor measured by independent medical physicists with
their own apparatus.
Material and Methods
The participants measured the output factor of the 5 mm
cone using a 6 MV photon beam at a source to surface
distance of 95 cm and depth in water of 5 cm. ARPANSA
provided a 3D scanning water tank for detector positioning
but all detectors were brought by participants. The
participant was asked to measure the output factor as
accurately as possible. All post measurement analysis,
correction factor determination and uncertainty
calculations were supplied by the participant.
Results
Fifteen groups travelled to ARPANSA and a total of thirty
independent measurements of the output factor were
made. The most popular method of measurement was with
film but measurements were also made with ionisation
chambers, semiconductor detectors, diamond detectors
and a scintillation detector. A large volume ionisation
chamber measuring dose area product was also used in the
comparison. The standard deviation of all the
measurements was 5.6 % with the maximum variation
between two results being 42 %.
Conclusion
This exercise gave an indication of the consistency of the
small-field dosimetry being performed in Australia at the
present time. There is no currently accepted protocol for
these measurements and a wide range of detectors are
being used with correction factors being applied from a
variety of sources. The dissemination of the small-field
methods and techniques currently being used will aid the
consistency of these measurements.
EP-1435 Evaluation of single material and multi-
material patient-specific, 3D-printed radiotherapy
phantoms
D. Craft
1
, E. Burgett
2
, R. Howell
1
1
The University of Texas MD Anderson Cancer Center,
Radiation Physics, Houston, USA
2
Idaho State Univeresity, Department of Nuclear
Engineering, Pocatello Idaho, USA
Purpose or Objective
Anthropomorphic phantoms are used in a variety of ways
in radiation therapy for both research and quality
assurance purposes. Most anthropomorphic phantoms are
of generalized patients, but 3D printing technology can be
used to fabricate patient-realistic phantoms for special QA
and verification procedures. Most 3D printers, however,
can only print in one or two materials at a time, so true
patient heterogeneity is limited. In this study, we
examined two different patient specific, 3D printed
phantoms created based on the same patient to determine
the accuracy of single and multi-material phantoms.
Material and Methods
The phantoms used in this study were designed from the
clinical CT data for a post-mastectomy patient treated at
our institution. The CT data was trimmed to remove the
patient’s head and arms to preserve anonymity and
simplify printing. Phantom 1 was designed by converting
the trimmed CT data into a 3D model with a CT threshold
of >-500 Hounsfield units (HU). This model was sliced into
2.5-cm-thick sagittal slices and printed one slice at a time.
All slices were printed with polylactic acid (PLA)
representing all body tissues, but with air cavities and
lower density regions like the lungs left open. Sagittal
slices were chosen for their superior fit with each other,
and minimal material warping relative to axial slices.
Phantom 2 was designed by converting the CT data into
three separate 3D models with a CT threshold of <-147 HU
for air cavities, -147 to 320 HU for soft tissue, and >320
HU for bone. The models were sliced into 1-cm-thick axial
slices, and printed. The slices were printed from the soft
tissue model using a custom formulated high impact
polystyrene (HIPS) with the air and bone models left open.
After printing, the open bone model sections were filled
with a liquid resin polymer with an equivalent density to
bone.
The phantoms were evaluated for their materials and
overall accuracy to the original patient CT. Blocks of PLA,
HIPS, and the bone resin material were all imaged to
determine their average HU. The phantoms were also each
imaged and registered with each other and the original
patient CT to determine the consistency and accuracy of
each phantom.
Results
The materials used and their properties are summarized
in Table 1. Phantom 1 was fabricated from PLA, which
isn’t particularly tissue equivalent, but did print relatively
consistently. The bone resin and HIPS of phantom 2 more
accurately reflect tissue heterogeneity, but have more
variations in their printed consistency.