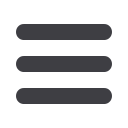

S792
ESTRO 36
_______________________________________________________________________________________________
tests makes it difficult to implement and results out of
tolerance are often left unexplained. Experimental
designs are a robust statistical method which minimizes
the number of tests to be performed and provides a
statistical analysis of the results. They were used to
compare computed and measured doses for several
algorithms.
Material and Methods
Tests were chosen using a Taguchi table L36 (2
11
x3
12
) to
enable the quantification of the influence of each
parameter. Five algorithms were studied: the AAA (version
11, Varian) is used in clinical routine and the collapsed-
cone convolution-superposition (CCCS) algorithm (version
1.5, Mobius Medical Systems) is used as a secondary dose
calculation plan check. The AcurosXB (AXB) algorithm
(version 11, Varian) was also investigated as well the
pencil beam (PB) and Monte Carlo (MC) algorithms
available on Iplan (version 4.5, Brainlab). Absorbed dose
was first calculated in water for 72 beams with varying
parameters: energy, MLC, depth, wedge angle, wedge
jaw, X, Y
1
and Y
2
dimensions. Computations were then
conducted for 72 beams in a CIRS Thorax phantom with
varying parameters: energy, wedge angle, wedge jaw, X
and Y dimensions, medium and gantry angle. Calculated
doses were compared to measurements conducted on a
Novalis TrueBeam STx (Varian) with a CC04 ionisation
chamber (IBA).
Results
In water, all algorithms gave a mean difference between
computed and measured doses centred on zero (within the
uncertainty). No studied parameter led to statistically
significant deviation. In the thorax phantom, the mean
difference between computed and measured doses was -
0.7 ± 1.1 % for AAA, -1.4 ± 1.4 % for CCCS, -2.5 ± 1.0 % for
AXB, 2.3 ± 2.2 % for PB and 0.3 ± 1.9 for MC. For AAA and
CCCS, calculations in bone medium led to a statistically
significant underestimation of the computed dose while
the other parameters had no influence on the results. For
MC, calculated dose was overestimated for gantry angle of
225° which was attributed to the modelization of the
treatment table by the TPS.
Conclusion
Experimental designs were used as a statistical method to
validate the AAA, CCCS and MC algorithms. The PB
algorithm was rejected for clinical use because it
overestimates the dose in heterogeneous medium. Results
showed that the AXB algorithm systematically
underestimates the dose in heterogeneous medium which
could be linked to the dose to water - dose to medium
conversion as referred in the literature. Further
investigation is needed before its implementation in
clinical routine, especially for modulated beams. The tests
described by the experimental designs were also used to
define the tolerance levels of the secondary plan check
software and are now part of the ongoing quality
assurance of the TPS
.
EP-1482 Signal Prediction for an On-line Delivery
Verification System
R. Heaton
1
, M. Farrokhkish
1
, G. Wilson
1
, B. Norrlinger
1
,
D.A. Jaffray
1
, M.K. Islam
1
1
Princess Margaret Cancer Centre University Health
Network, Radiation Physics, Toronto, Canada
Purpose or Objective
Dynamic radiation delivery techniques like VMAT
introduce challenges in treatment verification. Complex
treatments, as well as hypofraction and adaptive radiation
therapy, require new verification approaches to ensure
safe delivered. One approach is the introduction of
entrance fluence monitoring device, like the Integral
Quality Monitoring (IQM) System (iRT Germany), which
provides a spatially encoded dose area product signal as a
unique delivery fingerprint. Complementary to this
measurement is the signal calculation based on the
treatment plan. This work describes the calculation for
the IQM system and examines the impact of selected
components on clinical fields.
Material and Methods
The calculation models the spatial response of the IQM
chamber and the fluence transmitted through the
individual collimating elements. The chamber response is
modelled as a 2D map. The fluence from the machine is
divided into 2 components: a point source at the target
and an extended source at the flattening filter, referred
to as the primary and extended source, respectively. The
primary source is characterized by a radial intensity
profile and is attenuated through the jaws and multileaf
collimator. Transmission is calculated for a 2D array
matching the chamber response map, and area averaged
fluence is calculated for moving collimating elements
during beam delivery. The extended source is modeled as
a Gaussian distributed source with a Compton angular
intensity distribution. The contribution of the Gaussian
source to each element in the fluence array is raytraced
through the collimation to obtain the area averaged
fluence. An element-wise multiplication of the chamber
response map with the primary and extended source
fluence is summed to generate the predicted signal,
modified by factors reflecting the chamber volume, the
intensity of the primary and extended sources and change
in machine output with field aperture. The model has
been implemented for Varian and Elekta treatment units,
with calculations and measurements compared for
clinically relevant fields.
Results
Parameters for the model were determined from a series
of rectangular field measurements with the IQM chamber
combined with ion chamber measurements. Iterative
optimization of parameter values to match rectangular
field IQM measurement were performed. Similar
techniques were used to extract normalization
parameters.
The agreement between the calculated and measured
signals on a Varian TrueBeam unit for over 300 different
IMRT field segments from Prostate and Head & Neck plans
show 99% of segments agree within ±5%; 95% within ±3%.
Similar results were seen for an Elekta Agility unit in a
sample of over 400 different IMRT field segments, with
97% of segments agreeing within ±5% and 91% within
±3%.
Conclusion
A 2-source calculation model has been implemented for an
area-fluence monitor designed for on-line patient QA.
EP-1483 Pre-Treatment QA of MLC plans on a
CyberKnife M6 using a liquid ion chamber array.
L. Masi
1
, R. Doro
1
, O. Blanck
2
, S. Calusi
3
, I. Bonucci
4
, S.
Cipressi
4
, V. Di Cataldo
4
, L. Livi
5
1
IFCA, Medical Physics, Firenze, Italy
2
Saphir Radiosurgery Center, Medical Physics, Frankfurt/
Gustrow, Germany
3
University of Florence, Department of Clinical and
Experimental Biomedical Sciences "Mario Serio", Firenze,
Italy
4
IFCA, Radiation Oncology, Firenze, Italy
5
Azienda Ospedaliera Universitaria Careggi,
Radiotherapy Unit, Firenze, Italy
Purpose or Objective
CyberKnife MLC plans require accurate patient-specific
QA. The purpose of this study is to validate the use of a
liquid ion chamber array for Delivery Quality Assurance
(DQA) of robotic MLC plans, using several test scenarios
and routine patient plans and comparing results to film
dosimetry.