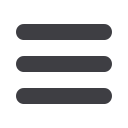

170
Chapter 8
striatum and a cortical region involved in attention processing, i.e. the intraparietal sulcus
(Padmala and Pessoa, 2011). This is illustrated further (and causally) in
chapter 7
, where we
show that manipulating the prefrontal cortex with TMS has effects on the integration across
task-related goals in the striatum without directly altering processing in the cortex. In fact,
activity in
chapter 4
was not restricted to the striatum, but was also revealed in the posterior
cingulate cortex, an area that is connected to the dorsal striatum (Di Martino et al., 2008;
Beckmann et al., 2009), suggesting indeed that processing in the corticostriatal network was
altered during motivated cognitive control in these patients.
The absence of significant effects in the striatum in studies that report prefrontal effects does
not necessarily imply that the striatum is not playing a role in the underlying process. Instead,
the measurement technique in studies that report effects in the prefrontal cortex may simply
not be sufficiently sensitive to detect changes in striatal BOLD response. The BOLD signal
in the ventral part of the brain, including the striatum is more susceptible to signal drop out.
Functional MRI may therefore generally be more sensitive to changes in signal in ‘cognitive’
dorsal cortical regions. Taking into account the
DAT1
genotype to account for inter-individual
differences in dopamine signalling in our work (e.g.
chapter 4
and (Aarts et al., 2010)) can
increase the sensitivity to detect changes in the striatum.
These studies are generally in line with the idea that reward changes processing in the cognitive
control region involved in the task at hand. And they suggest that the prefrontal cortex and
striatumcan act in concert tomediate the interaction between different task aspects. However,
in
chapter 1
we argued and illustrated that reward motivation can have opposite effects on
cognitive flexibility and stability and we argued that this effect may be due to opposing effect
of dopamine on cognitive flexibility and stability (Durstewitz and Seamans, 2008). The idea
that dopamine can be detrimental for cognitive focussing was demonstrated recently in a
study using a Stroop task (Aarts et al., 2014b), for which good task performance requires a
stable task representation (i.e. not be distracted by the irrelevant dimension in the incongruent
condition). Importantly, rewarded Stroop performance was associated with dopamine levels
(i.e. dopamine synthesis capacity). More specifically, increased dopamine levels in this study
were associated with a detrimental effect of reward on cognitive performance. The work in
this thesis focused on cognitive flexibility, and did not formally manipulate stability. However,
whereas one may think of switch trials as a form of cognitive flexibility, performance on repeat
trials will likely benefit from stable task representations. The results in
chapter 5
revealed
opposing age-related effect of reward motivation on switch and repeat trials, suggesting
that (reward and age-induced changes in) dopamine can have opposite effects on cognitive
flexibility and cognitive focussing (
limitations
). The number of studies that assessed the
role of striatal dopamine in mediating the integration between reward and cognitive control
(whether it is flexible control or maintaining stable task representations) is scarce. Therefore
it remains unclear which factors determine whether the effects of dopamine are beneficial or
detrimental for cognitive control. Reward-induced changes in dopamine may modulate how
susceptible the cognitive (dorsal) striatum is for input from the reward (ventral) striatum or