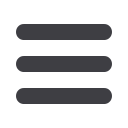

S487
ESTRO 36
_______________________________________________________________________________________________
PO-0887 Experimental validation of a 3D model to
simulate FMISO spatial retention in HNSCC tumor
xenografts
L.J.M. Wack
1
, A. Menegakis
2
, R. Winter
1
, S. Boeke
2
, K.
Trautmann
3
, A. Leun
1
, M. Krueger
4
, B. Pichler
4
, D.
Mönnich
1
, D. Zips
2
, D. Thorwarth
1
1
Clinic for Radiation Oncology- University Hospital
Tübingen, Section for Biomedical Physics, Tübinge n,
Germany
2
University Hospital Tübingen, Department of Radiation
Oncology, Tübingen, Germany
3
University Hospital Tübingen, Department of Pathology
and Neuropathology, Tübingen, Germany
4
Preclinical Imaging and Radiopharmacy, Werner Siemens
Imaging Center, Tübingen, Germany
Purpose or Objective
Tumor hypoxia is prognostic for poor outcome after
radiotherapy (RT). A method for non-invasi ve assessment
of hypoxia is PET using hypoxia radiotracers such as FMISO.
For this study, we evaluated a tool to simulate 2D and 3D
FMISO accumulation on realistic vessel architectures,
which can be compared against experimental
pimonidazole (pimo) stainings of the same tumor.
Material and Methods
Dynamic PET/MR imaging was performed in FaDu tumors
(human HNSCC) grown in the right hind leg of nude mice
for about 5 weeks, using an injected FMISO activity of
~12MBq. Pimo and hoechst 33342 were injected 1h and
1min prior to tumor excision, respectively, to allow
staining for tumor hypoxia and perfusion status of blood
vessels. After excision, two tumors were snap frozen and
the central part was cut into 120 consecutive sections of
10µm. Immunofluorescence staining was performed for
pimo and endothelial marker CD31. Sections were
subsequently scanned on a Zeiss Axiovert fluorescence
microscope to detect pimo, CD31 and hoechst. The
fluorescence images were rigidly registered, manually
adjusted and thresholded to create a binary 3D vessel map
(VM). Hoechst-negative vessels were excluded from the
VM. These VMs were used to simulate 3D oxygen
distributions based on a Michaelis-Menten relation. An
average input function (AIF) was determined by fitting
activities in the left ventricles over 4 mice to derive mean
parameters. Based on oxygen distribution and AIF, FMISO
retention was simulated on the same VMs. FMISO-positive
regions of 3x3mm2 in the tumor center in 5 random
sections were compared against manually contoured
pimo-positive regions to validate the simulation by
determining hypoxic fraction (HF) and overlap ratio.
Necrosis was excluded based on H/E staining on the same
sections. To compare 3D and 2D simulations, the
simulations and analysis were repeated in 2D. Parameters
for all simulations were set to commonly used values
(Mönnich et al., 2011).
Results
Differences in experimental and 3D-simulated hypoxic
fractions (HF) were not significant, while differences
between experimental and 2D-simulated HF was
significantly different for Tumor 2 (p=0.02, cf. Table).
3D simulations matched much better with pimo
distribution than 2D simulations only. The true-positive
rate was increased about 0.2 for both tumors, the true-
negative rate by about 0.08 for 3D simulations when
compared to 2D. 56% of 3D-simulated FMISO-positive
voxels were located within pimo-positive areas, while
another 14% were located within 50µm distance, as to 37%
and 8% for 2D, respectively (cf Table, Figure).
Conclusion
When performing hypoxia tracer simulations on actual
VMs, 3D models accounting for out-of-plane diffusion must
be used to obtain realistic results. In a 3D vascular model,
spatial tracer distributions similar to those observed
in
vivo
can be simulated. Hence, 3D FMISO simulation on