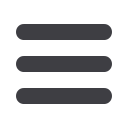

S777
ESTRO 36 2017
_______________________________________________________________________________________________
characteristics (80 or 120 leaves) are indicated.
Fig 1. Dose volume histogram for the whole body averaged
over the 10 patients of this study, comparing every
treatment technique.
Conclusion
The source for higher values of ID and NTID for HT is the
larger volume receiving dose below 20 Gy. No differences
were found in the election of IMRT delivery. For RapidArc
plans, ID and NTID values are similar to IMRT.
EP-1472 Dosimetric E2E verification using 3D printing
and 3D dosimeter for brain stereotactic radiotherapy
M.S. Kim
1
, K.H. Chang
1
, J. Kwak
1
, G.M. Back
1
, T.Y. Kang
1
,
S.W. Kim
1
, Y. Ji
1
1
Asan Medical Center- Univ of Ulsan, Radiation Oncology,
Seoul, Korea Republic of
Purpose or Objective
To evaluate the dosimetric accuracy of brain stereotactic
radiotherapy (SRT) with a 3D dosimetry system and MRI,
we investigated dosimetric end-to-end verification using
3D printing technology and 3D dosimeter.
Material and Methods
We implemented an anthropomorphic head and neck
phantom with a 3D printed insert made using a 3D printer
designed by the Autodesk software and two gel-filled
spherical glass flasks as a patient having multiple target
brain cancer. For the feasibility study of the gel
dosimeter, the dose linearity, dose rate dependence, and
reproducibility for the gel dosimeter were verified. Gel-
filled vials were irradiated with 6 MV beams to acquire a
calibration curve of dose relation to R2 (1/T2) values in
9.4T MR images. Graded doses from 0 to 8 Gy with an
interval of 2 Gy were delivered. Two PTVs (PTV1,2) were
contoured on the MR images of phantom have dosimetric
gel tumor. To evaluate geometric and dosimetric
accuracy, a treatment plan was created such that D95s for
PTV1 and intentional PTV2 were more than the prescribed
dose. The intentional PTV2 was produced by intentionally
shifting by 5mm from the true target position. 2 arc VMAT
plan was created to deliver 35 Gy in 5 fractions. After
irradiation, calibration vials and phantom were scanned
by 9.4T MRI and then acquired images were analyzed using
an ImageJ and DCMTK software libraries. Scanned MRI
images of phantom were imported to a treatment planning
system and registered to CT images to compare dose
distributions. We also compared the agreement result
between the planned and the measured data in 1D (ion
chamber), 2D (gafchromic film), and 3D (Gel dosimeter).
Results
The best dose linearity was 0.99 (R
2
) at 180 TE (ms).
Reproducibility and dose rate dependency were less than
2.2% and 3.5%, respectively for 180 TE. Point dose
differences in plan vs. ion chamber were 1.08%, 0.47%,
and -2.82%, respectively, for PTV 1, 2, and intentional
PTV. And its differences between plan and gel were 0.98%,
1.66% and 3.76%, respectively, for PTV 1, 2, and shifted
PTV. Gamma passing rates with 3%/3mm criteria were
greater than 99% for all plans. Isodose distributions and
profiles showed qualitatively good agreement between
the gel dosimeter, EBT film and RTP data for all PTVs.
Conclusion
The results indicate that those processes could effectively
evaluate geometric and dosimetric accuracy of brain SRT.
This study using 3D dosimetry system was useful to
validate the 3D dose distributions for patient-specific QA.
EP-1473 Improving the accuracy of dosimetry
verification by non-uniform backscatter correction in
the EPID
Y. Md Radzi
1,2
, R.S. Windle
2
, D.G. Lewis
2
, E. Spezi
1,2
1
Cardiff University, School of Engineering, Cardiff,
United Kingdom
2
Velindre Cancer Centre, Department of Medical Physics,
Cardiff, United Kingdom
Purpose or Objective
Challenges in improving the accuracy of EPID-based
patient dose verification have been widely discussed and
remain a key topic of interest for patient safety, as
exemplified in the UK by the ‘Towards Safer Radiotherapy’
2008 report[1]. In particular, one of which is for every
radiotherapy centre to have protocols for in vivo
dosimetry (IVD) to be used for most patients as
recommended in the Annual Report of the Chief Medical
Officer for 2006 and it is already a legal requirement in
many European Countries [2]. In this presentation, we
report on commissioning and implementation of the
commercially available Dosimetry Check (DC) [3, 4]
system. Particular emphasis has been given to addressing
the significant non-uniform backscatter effect from the
VARIAN aSi-1000 EPID arm [5, 6].
Material and Methods
A backscatter correction matrix was developed by
combination of dosimetric information from a set of
segmented fields sampling on different positions around
the active area of the imager. The matrix was then used
to correct EPID images using MATLAB programming scripts.
The corrected image was created in DICOM format and
exported to Dosimetry Check to read and analyse.
Example treatment fields were generated in our Oncentra
MasterPlan (OMP) Treatment Planning System (TPS), with
several equidistant dose reference points relative to
central axis included. A dose comparison given by DC with
reference to the TPS was recorded in an auto-generated
report. Assessment and comparison undertaken included
the
(i)
asymmetry evaluation of equidistant points before
and after correction being applied with respect to TPS,
(ii)
improvement in segmented IMRT dose profiles after
correction, and
(iii)
OMP-DC pass rate with gamma
criterion 3%/3mm[7], as well as 2-D Gamma Volume
Histogram (GVH) evaluation on outlined PTVs.
Results
(i)
Correction for non-uniform backscatter improved with
overall agreement between fields generated in OMP and
those recorded in DC from within 3% to better than 1%.
(ii)
Agreement between OMP and DC for IMRT dose profiles
with a sample Head & Neck case was improved by
approximately 3% using the correction methodology
(
Table 1
).
(iii)
For gamma comparison of fields in OMP and
DC with 3%/3mm, pass rates were improved from around
80% to around 90% by the correction method. Similarly in
GVH evaluation for the outlined PTVs, pass rate has
increased from around 80% to 90% after correction being
applied.