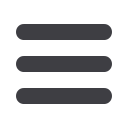

S780
ESTRO 36 2017
_______________________________________________________________________________________________
patient’s -5° gantry error was deemed unacceptable and
subsequently measured, patient 2 with this error detected
(gamma pass rate of 68.8%).
Results
The results for 10 patients are shown in Figure 1.
Figure 1. Gamma pass rate (%) for non-error (NE) plans
and for deliberately introduced errors (including the
Collimator (C), MLC shift (S), and MLC Field Size (F)
error), where the latter are selected as exceeding dose
tolerances by the smallest magnitude. *Patient 1 F error
of -5 was not deliverable due to machine tolerances,
hence an F error of -2 is utilised here.
The global 3%/3mm gamma pass is able to detect the
majority of unacceptable plans, however some MLC field
size plans still pass. Decreasing the MLC field size by 1mm
can result in significantly reduced dose to the PTV, which
affects tumour control e.g. patient 3 MLC FS-1 passed,
however this plan under-doses the PTV63Gy by -5.4%
relative to the original non-error plan.
Conclusion
Not all deliberately introduced clinically significant errors
were discovered for VMAT plans using a typical 3%/3mm
(10% threshold with correction off) gamma pass rate.
EP-1478 A split field beam model of Beam Modulator
linear accelerator in Pinnacle treatment planning
system
M. Chandrasekaran
1
, S. Worrall
1
, M.K.H. Chan
1
, N.
Khater
1
, C. Birch
1
1
University Hospital Southampton NHS Foundation Trust,
Radiotherapy Physics, Southampton, United Kingdom
Purpose or Objective
The aim of this study was to model a beam modulator
linear accelerator in Pinnacle v.14.0 treatment planning
system for intracranial stereotactic radiosurgery and
radiotherapy.
Material and Methods
Depth dose, beam profile and total scatter correction
factor data were collected for 6 MV photons of Elekta
Synergy Beam Modulator
TM
linear accelerator with 80
leaves each of 4 mm leaf pitch using unshielded IBA
stereotactic field diode for field sizes ranging from 0.8x0.8
cm
2
to 4x4 cm
2
and field sizes above 4x4 cm
2
up to 16x21
cm
2
using IBA CC04 pinpoint chamber. The measured data
were imported in to the photon physics module of Pinnacle
v.14.0 and physical accelerator head specific data such as
primary collimator, flattening filter, MLC were input in
addition to beam data measurements. The auto modeler
of Pinnacle TPS was iteratively used to adjust parameters
such as photon beam energy spectrum, Gaussian height
and width of the photon source that affect various regions
of the depth doses and beam profiles to match measured
data. Dose grids of 1 mm and 2.5 mm were used for beam
modelling of fields from 0.8x0.8 cm
2
up to an equivalent
square field of 6.7 cm
2
and above 6.7 cm
2
respectively. A
common photon energy spectrum did not prove sufficient
to achieve the required agreement between Pinnacle
calculated and measured depth doses and beam profiles
for the whole range of field sizes. This was overcome by a
split field model that employs field size specific beam
energy spectra, with higher relative weights of low energy
bins and lower relative weights of high energy bins for
small fields and vice-versa for field sizes larger than 6.7
cm
2
. The validity of the model was tested independently
using a Standard Imaging Exradin A26 chamber in LUCY
phantom for field sizes ranging from 0.8x0.8 cm
2
by
comparing calculated and measured absolute doses and
relative output factors.
Results
Optimization of photon beam energy spectrum specific to
small field sizes improved the agreement of depth doses
both in and beyond build-up region for the small fields.
Measured versus calculated absolute planned doses were
found to be within 1% for field sizes larger than 1.6x1.6
cm
2
and less than 2.5% for 0.8x0.8 cm
2
field. The
agreement between the measured and calculated relative
output factors were within 2% for field sizes larger than
1.6x1.6 cm
2
and less than 3.5% for 0.8x0.8 cm
2
fields.