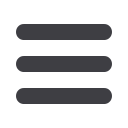

S406
ESTRO 36
_______________________________________________________________________________________________
PO-0767 Revisiting EPID design for modern
radiotherapy requirements
P. Vial
1,2
, S. Blake
2,3
, Z. Cheng
2,3
, S. Deshpande
1,4
, S.
Atakaramians
5
, M. Lu
6
, S. Meikle
7
, P. Greer
8,9
, Z. Kuncic
2
1
Liverpool and Macarthur Cancer Therapy Centres and
Ingham Institute, Department of Medical Physics,
Liverpool BC, Australia
2
University of Sydney, Institute of Medical Physics-
School of Physics, Sydney, Australia
3
Ingham Institute, Medical Physics, Liverpool, Australia
4
University of Wollongong, Centre for Medical Radiation
Physics, Wollongong, Australia
5
University of Sydney, Institute of Photonics and Optical
Science- School of Physics, Sydney, Australia
6
Perkin Elmer, Medical Imaging, Santa Clara, USA
7
University of Sydney, Faculty of Health Sciences & Brain
and Mind Centre, Sydney, Australia
8
University of Newcastle, School of Mathematical and
Physicsal Sciences, Newcastle, Australia
9
Calvary Mater Newcastle Hospital, Radiation Oncology,
Newcastle, Australia
Purpose or Objective
New methods of treatment verification that are in keeping
with advances in radiotherapy technology are desirable.
The availability of kilovoltage in-room imaging for
example has led to a general trend away from the poorer
contrast megavoltage (MV) imaging for patient-set-up.
The widespread use of intensity-modulated radiotherapy
(IMRT) also reduces the utility of treatment beams as a
source of imaging for treatment verification. At the same
time there has been a steady increase in the use of
electronic portal imaging devices (EPIDs) for dose
verification. There is however emerging evidence of new
roles for MV imaging in real-time target tracking. In this
work we address the issue of EPID detector specifications
in light of changing clinical requirements. We present a
general overview of the detector development work our
group has undertaken to design an EPID that better
supports applications relevant to current and future
clinical practice.
Material and Methods
Prototype EPID technologies developed by our group
include: a direct detector EPID where the metal/phosphor
screen has been replaced by a water equivalent build-up
material [1]; a dual detector combining a standard EPID
and an array dosimeter [2]; and an EPID comprising a
plastic scintillator fibre array (PSFA) in place of the
metal/phosphor screen [3]. Our performance
specifications were to achieve imaging performance
equivalent to standard EPIDs, and a dose response
equivalent to standard clinical dosimeters. Quantitative
metrics such as detective quantum efficiency (DQE) for
imaging and field size response for dosimetry were used in
both experimental and Monte Carlo (MC) studies. There
are three arms to this project that shall be described; i)
MC simulations to characterise and design scintillators, ii)
Prototype construction and experimental evaluation, iii)
clinical implementation.
Results
All prototype detectors exhibited near equivalent dose
response with ionisation chambers in both non-transit and
transit geometries (± 2%), including 2D clinical dosimetry
of IMRT fields. The X-ray quantum efficiency of the direct
and PSFA detectors is approximately 9% compared to 2%
for the standard EPID and dual detector. The imaging
performance of the standard EPID and dual detector
remains superior to the other prototypes because of the
greater efficiency of optical photons detected per
incident X-ray and better spatial resolution. MC
simulations demonstrate potential improvements in
imaging with the PSFA. A model for clinical
implementation has been developed that exploits the
water equivalence of the detectors. A water equivalent
EPID provides more direct and robust verification than can
be achieved with current EPID dosimetry. A water
equivalent EPID that retains imaging capability is better
suited than current EPIDs for modern radiotherapy.
Conclusion
This work demonstrates the feasibility and advantages of
alternative EPID designs that better meet the needs of
modern radiotherapy.
PO-0768 Electron Paramagnetic Resonance signal from
a new solid polymer material aimed for 3D dosimetry
M.R. Bernal-Zamorano
1
, N.H. Sanders
1
, L. Lindvold
1
, C.E.
Andersen
1
1
DTU, Nutech, Roskilde, Denmark
Purpose or Objective
We have developed a water-equivalent solid polymer
dosimeter material aimed for 3D dosimetry in
radiotherapy beams. The material responds to ionizing
radiation by changes in its optical absorbance and by
generation of fluorescence centers. The latter signal is of
particular interest as the fluorescence centers facilitate
detailed mapping the 3D dose distribution us ing laser
stimulation. However, in addition to the optical si gnals
we also expect that the material could have an electron
paramagnetic resonance (EPR) dose response related to
the production of stable free radicals. To test this
hypothesis, point detector experiments were therefore
performed where the material was casted into 5 mm
diameter pellets identical in size to the alanine dosimeters
that we routinely use for reference EPR dosimetry in our
laboratory. The pellets of the new material and alanine
were irradiated in
60
Co beams and EPR signals were
recorded afterwards.
Material and Methods
The dosimeter is based in pararosaniline leuco dye, which
is chemically transformed into its dye-form by the effect
of radiation. The leuco dye is dissolved in a poly(ethylene
glycol) diacrylate matrix (PEGDA-575 g/mol) that contains
diphenyl(2,4,6-trimethylbenzoyl)phosphine oxide (TPO)
used for photocuring. We cured the material in a mold
with a 395 nm LED for a few minutes. We made 4
cylindrical pellets of 4.75 mm diameter and 2.78 mm
thickness (same size than alanine dosimeters used in this
work).
Pellets of the new material and alanine were irradiated in
a
60
Co gamma source with a dose rate of about 8 Gy min
-1
.
They were given doses of 5, 10, 20, 30, 50, 75 and 100 Gy.
The EPR signal for both dosimeters was obtained by a
Bruker EMX-micro spectrometer by inserting the pellets
into the resonator in a quartz tube. Absorbance and
fluorescence signals of the pellets of our material were
measured with a Shimazdu UV-2700 spectrophotometer
and an Ocean Optics QE6500 spectrometer respectively.
Fluorescence was excited with a diode laser.
Results
A clear EPR signal was obtained for our material, and this
signal increased with dose. The peak-to-peak amplitude of
the EPR spectra are shown in the figures.
Although alanine and PEGDA have similar characteristics
in terms of its water equivalence (similar effective atomic
number, mass density and electronic density), their EPR
signal is very different.