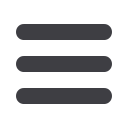
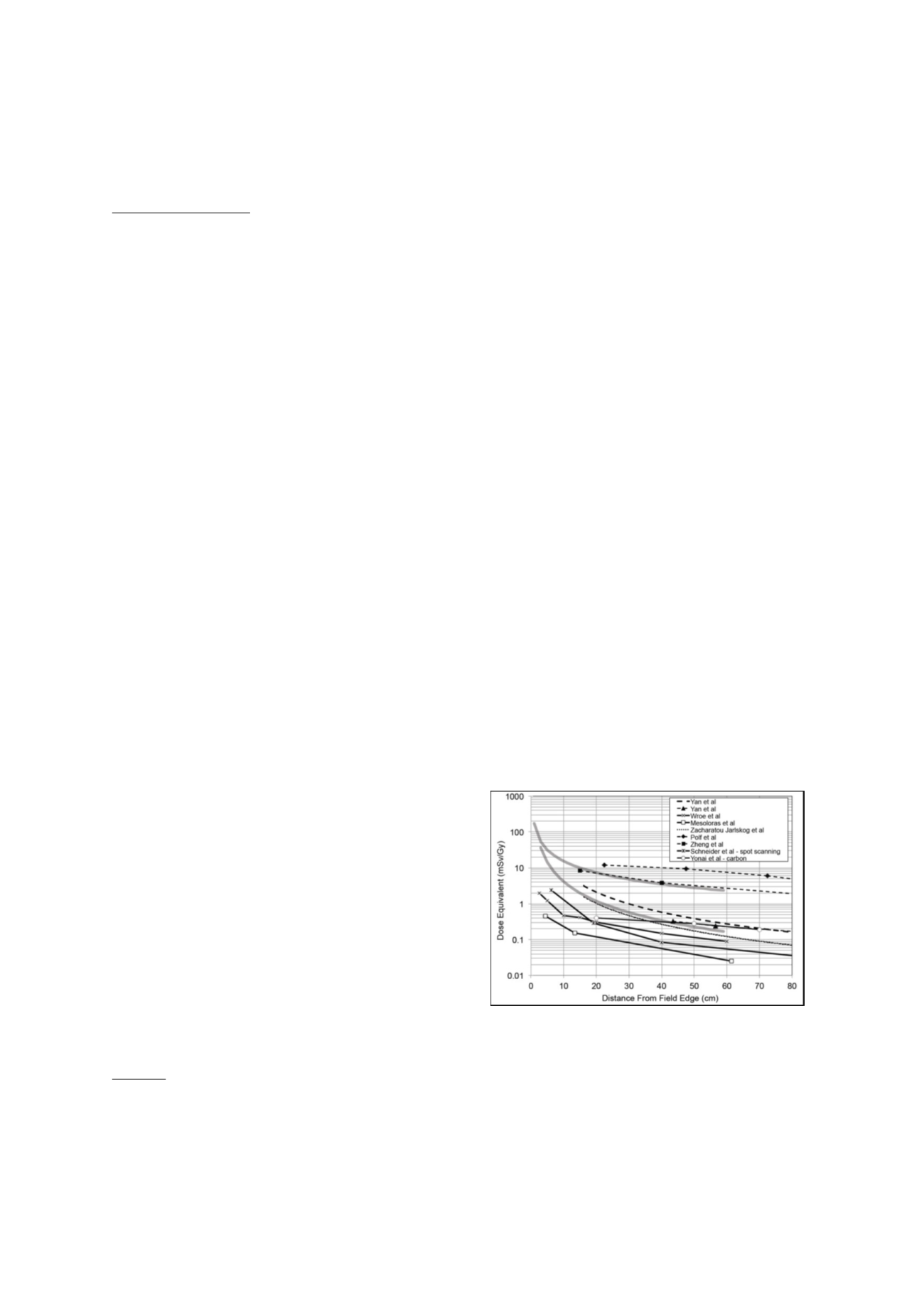
S221
ESTRO 36 2017
_______________________________________________________________________________________________
obtained are instrumental for building and validating
beam models for MRiPT, as reported in a separate
abstract.
PV-0422 Direct determination of kQ in a clinical
carbon ion beam using water calorimetry
J.M. Osinga-Blättermann
1,2
, U. Ankerhold
1
, S. Brons
3
, S.
Greilich
2
, O. Jäkel
2,3
, A. Krauss
1
1
Phys. Techn. Bundesanstalt PTB, Department of
Dosimetry for Radiation Therapy and Diagnostic
Radiology, Braunschweig, Germany
2
German Cancer Research Center DKFZ, Division of
Medical Physics in Radiation Oncology, Heidleberg,
Germany
3
Heidelberg Ion-Beam Therapy Center, HIT, Heidelberg,
Germany
Purpose or Objective
Until now, the dosimetry of carbon ions with ionization
chambers has not reached the same level of accuracy as
that of high-energy photons: the associated standard
uncertainties differ by about a factor of three [TRS-398,
IAEA, 2000]. This is mainly caused by the limited
knowledge of the so-called
k
Q
factor, which corrects for
the different response of the ionization chamber to the
actual user beam quality
Q
(here:
12
C) compared to the
reference beam quality
Q
0
(here:
60
Co). The aim of this
work is to experimentally determine the
k
Q
factor in order
to exploit the possibility of significantly improving the
accuracy of ionization chamber-based dosimetry of
clinical carbon ion beams.
Material and Methods
Water calorimetry by means of the transportable water
calorimeter of the National Metrology Institute of
Germany (PTB - Physikalisch-Technische Bundesanstalt) is
implemented in the entrance channel of a scanned 6 cm
x 6 cm radiation field of 429 MeV/u carbon ions at the
Heidelberg Ion-Beam Therapy Center (HIT). This enables
the direct calibration of ionization chambers and thus the
experimental determination of
k
Q
. In order to achieve an
overall low measurement uncertainty, the irradiation
parameters and the resulting radiation field have been
characterized in detail as they strongly influence several
calorimetric and ionometric correction factors. In total,
three separate series of measurements were performed to
determine the values for
k
Q
for the two Farmer-type
ionization chambers FC65-G (IBA) and TM30013 (PTW).
Results
By means of water calorimetry, a standard measurement
uncertainty of 0.8% could be achieved for the
experimental
k
Q
values corresponding to about a threefold
reduction of the uncertainty compared to calculated
values. For both ionization chambers, a comparison of the
experimental
k
Q
factors with corresponding literature
values (TRS-398, German DIN 6801-1) will be presented
and discussed.
Conclusion
This study showed for the first time that the experimental
determination of the
k
Q
factor for carbon ion beams by
means of water calorimetry is achievable with
unprecedented accuracy. This result enables the
significant reduction of the overall uncertainty related to
ionization-based dosimetry of clinical carbon ion beams.
PV-0423 AAPM TG-158 recommendations for neutron
dosimetry for photon, electron, and light-ion therapy.
R. Howell
1
, B. Bednarez
2
, S. Kry
1
1
UT MD Anderson Cancer Center Radiation Physics,
Radiation Physics, Houston- TX, USA
2
University of Wisconsin, Medical Physics, Madisson, USA
Purpose or Objective
The American Association of Physicists in Medicine Task
Group (TG) 158, measurement and calculation of doses
outside the treatment volume from external-beam
radiation therapy (EBRT), was created to provide guidance
for physicists in assessing and managing non-target
doses. Neutron detection in particular, presents many
unique challenges and is infrequently performed by
medical physicists. Neutron data in the literature span
many orders of magnitude and are difficult to interpret
and compare. The primary objectives of this presentation
are convey the neutron relevant information from TG-158
for photon, electron, and light-ion EBRT: (1) to provide an
overview of neutron data reported in the literature (2) to
summarize various detectors that can be used to measure
secondary neutrons and specifically address limitations in
different measurement environments and (3) summarize
recommendations from AAPM TG-158 for neutron
dosimetry for clinical care and research applications.
Material and Methods
The TG-158 was completed in 2016 and is expected to be
published in 2017. The committee reviewed
approximately 320 publications in the litera ture, a large
fraction of which focused on secondary neutrons and
neutron measurement techniques for photon, electron,
and light-ion EBRT.
Results
This presentation will provide an overview of neutron data
reported in the literature, which span m any orders of
magnitude. Figure 1 is an example of neut ron data from
proton and carbon therapy. Similar examples will be
discussed for photon and electron beam EBRT. This
presentation will also summarize various detectors that
can be used to measure secondary neutr ons. Neutron
detectors are highly energy dependen t and thus,
knowledge of the energy spectrum being measured is
essential. The secondary neutrons from electron, photon,
and light-ion therapy have a wide energy range, i.e., from
thermal up to about 10 MV for photon/electron therapy
and thermal up to 250 MeV for light ion
therapy. Moreover, many neutron detectors cannot be
used in or near the primary field because of issues such as
pulse-pile-up and interactions of particles within the
detector, among others. Thus, each neutron detector will
be presented in the context of its energy sensitivity and
its suitability for measurements in-or near the primary
field. Finally, this presentation will summarize the
recommendations of TG-158 for neutron dosimetry for
clinical
care
and
research
applications.
Figure 1:
Summary of published data from several studies
of neutron dose equivalent from proton and carbon
therapy. The upper and lower bounds of neutron dose
equivalent from photon IMRT data are also included for
reference (solid grey lines).
Conclusion
This presentation will highlight the unique challenges of
measuring neutrons and will provide guidance on how to
select the most appropriate for these measurements for
photon, electron, and light-ion therapy.