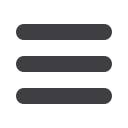

March 2015
AFRICAN FUSION
27
obtained for the interlayer and the 27NiCrMoV base material
respectively. Local maxima were again found in heat affected
zones – the highest in HAZ of 27NiCrMoV base material (up to
350 HV near the face of weldment), lower in the HAZ of the
interlayer (up to 300 HV) and about 320 HV in HAZ of COST F
base material. This means that carbon enrichment increases
hardness, yet the increase is not essential for macroscopic
mechanical properties. The interlayer was again the weakest
part of the weldment.
Dissimilar weld joint D
The last trial weld joint investigated, the weldment of COST
F and COST FB2 steels, was produced in two variants with
different weld metals – Thermanit MTS 3 (D1 weld joint) and
Thermanit MTS 616 (D2 weld joint), which are both based on
modified 9Cr steels. Thermanit MTS 3 contains 1.0 wt.% of
molybdenum, while Thermanit 616 contains 0,4 wt.% of mo-
lybdenum and 1.7 wt% of tungsten. Each variant underwent
three different heat treatment schedules. The best one was
selected on the basis of results of mechanical testing and a
detailed microstructural study was performed using LM, SEM
and TEM. Using that optimised technology a new weld joint
was produced and long term creep testing was carried out.
Results of creep testing together with fractographic analysis
and TEM of carbon replicas and thin foils are summarised in
another paper of this proceedings (Kasl J, Jandov
á
D:Testing
of dissimilar weld joint of steels COST FB2 and COST F).
Microstructures in all zones of theweldment corresponded
to heavy tempered martensite with a high density of precipi-
tates (Figures 13 to 15). The COST F base material unaffected
by welding was significantly coarser than that of FB2. Small
islands of
δ
-ferrite were sporadically observed in the base
materials and more often in the weld metal, especially in the
root. Density of precipitates decreased in the sequence from
FB2 to COST F base materials and then to the weld metal. No
differences between D1 and D2 variants were observable us-
ing LMand SEM. The variant with the weldmetal of Thermanit
616 showed better mechanical properties; therefore this one
underwent additional microstructural study and testing under
creep conditions.
Crossweld hardness profiles of all six variants of weld D
weremeasured (twoweld joints after threemethods of PWHT).
The hardness of the D2 weld after optimal heat treatment
is shown in Figure 16. The hardness of both base materials
unaffected by welding is approximately the same – about
240 HV 10 – and decreased in the HAZ, especially in FB2 steel
to around 200 HV 10. Higher hardness (up to 280 HV 10) was
found in the weld metal. This hardness profile corresponded
to results fromtensile testing. All specimens ruptured in either
the FB2 or COST F base materials.
References
1
Kern TU, Mayer KH, Donth B, Zeiler G, Digianfrancesco
A: The European Efforts in Development of New High
Temperature Rotormaterials – COST536; Proceeding of
the 9
th
Liege Conference, Materials for Advanced Power
Engineering, 2010.
2
J Lecomte-Beckers, Q Contrepois, T Beck, B Kuhn Eds:
Forschungzcentrum J
ü
lich GmbH, Proceeding of the
9
th
Liege Conference, Materials for Advanced Power
Engineering, 2010. pp. 27-36.
Conclusions
Four trial weld joints were investigated. Microstructure was
evaluated using light, scanning and transmission electron
microscopy and thesewere comparedwith results ofmechani-
cal testing. It then became possible to optimise consumables
and PWHT as well as heat treatments of the base materials.
Full conditions of the heat treatments of individual weld joints,
however, cannot be published.
After qualification tests and finalisation of the weld joint
designs, these procedures will be used in real production of
combined rotors for turbines for fossil fuel power plants
Acknowledgements
This work was supported by Grant project TE01020118 from
the Technology Agency of the Czech Republic.
Figure 12: The crossweld hardness profile of Weld C after PWHT.
Figure 13: LM micrograph of Weld D2
showing a martensitic structure in the
COST FB2 base material.
Figure 14: LM micrograph of Weld
D2, which also reveals a martensitic
structure in the COST F base material.
Figure 15: LM micrograph of Weld D2
showing a martensitic structure in the
weld metal.
Figure 16: Crossweld hardness profile of Weld D after optimal PWHT.